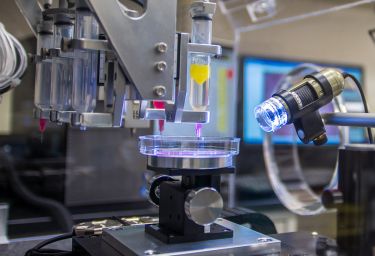
Health & Medicine
Five ways 3D printing is changing medicine
A new way to 3D print human tissue is revolutionising how we create and test medical treatments
Published 31 October 2024
3D printing has exploded in popularity over the past decade, introducing countless new approaches that allow both researchers and hobbyists to transform their designs into physical objects in just a few hours – far quicker than the days or weeks it used to take.
This shift in manufacturing has also been revolutionary for biologists, opening the door to creating complex three-dimensional scaffolds that mimic the tissues and organs in our bodies.
Bioprinting, a breakthrough field, means scientists can now move beyond the basic 2D cell cultures that have dominated biological research for decades.
Most 3D bioprinters use a movable syringe filled with a slurry of cells and biocompatible polymers. The syringe squeezes out these cells and polymers to form a shape.
By stacking these shapes on top of each other, researchers can gradually build up a 3D object that imitates tissues like bone, cartilage or even brain matter.
Health & Medicine
Five ways 3D printing is changing medicine
More recent methods use liquid polymers that harden when exposed to light to define each layer, but the general principle remains the same: structures are built up layer-by-layer in a slow, step-by-step process.
The printed objects are typically attached to a solid base or platform and need to be mechanically removed from the base once the 3D shape is complete.
One of the major challenges of bioprinting is working with living cells, which require a precise balance of nutrients, chemical signals and environmental conditions to thrive and grow into the complex tissues found in our bodies.
Meeting these strict conditions is a real challenge in bioprinting, so our team in the Collins BioMicrosystems Laboratory, led by Associate Professor David Collins in the Department of Biomedical Engineering, pioneered a new method that allows us to print objects directly into a liquid environment – a technique that is significantly faster and more suitable for fabricating delicate tissues.
Working with colleagues from the ARC Centre of Excellence for Transformative Meta-Optical Systems (TMOS), The Florey and Royal Melbourne Hospital, we’ve developed a new technique called Dynamic Interface Printing (DIP).
Recently published in Nature, DIP takes a new approach to bioprinting – eliminating the need for a solid platform.
This allows us to fabricate tissues in common lab objects like Petri dishes, vials, and multi-well plates – which are ideal for conducting further biological analysis.
One of the key advantages of DIP is that it removes the often-damaging step of detaching the object from a hard surface, which is especially crucial when recreating sensitive tissues like brain matter or body fat.
In DIP, a bubble forms beneath the liquid polymer, meaning the object is supported by the surrounding fluid.
This process means complex structures can be printed in seconds, pushing the boundaries of what’s possible in tissue engineering.
Sciences & Technology
We’re closer to ‘engineering’ blood vessels
The cells that make up tissues and organs in our bodies must work in harmony to function properly – a bit like an orchestra.
With a wave of the conductor’s baton, each musician is guided to play their part at the correct tempo and volume, producing a beautiful symphony.
To recreate this harmony in bioprinting, we use sound waves to vibrate the bubble, producing ‘ripples’ across its surface, just like when a pebble is dropped in a pond.
By precisely controlling these ripples, we can generate pressure fields that guide cells into specific, three-dimensional arrangements during the printing process.
Until now, arranging cells into well-defined 3D patterns has been very difficult. But with our new approach, not only can we position cells with precision, we can also fabricate at a scale of single cells.
This breakthrough represents the next generation of biofabrication technology, giving researchers and clinicians the tools to create increasingly complex and representative tissue models with unprecedented accuracy.
While 3D-printed organs are still a bit futuristic, we are getting much closer to some innovations that could drastically improve the way we create new drugs and treat illnesses.
As we move towards more sophisticated tissue models derived from human cells, we have the potential to reduce our reliance on animal testing.
Health & Medicine
The genomic jigsaw of cancer
These tissue mimics are poised to more accurately replicate human biology, allowing researchers to better predict how drugs will behave in the human body.
This not only promises to improve drug safety and effectiveness but to also accelerate the development of treatments by offering more reliable preclinical testing platforms.
Our DIP system is beginning to bridge the gap between small-scale lab experiments and the larger-scale needs of clinical diagnostics.
This means that the current ‘one-size-fits-all’ approach to disease treatment could soon become obsolete.
Determining the right treatment for a patient – especially those who are immunocompromised – can be incredibly challenging, involving a lot of trial and error and potentially causing unnecessary harm through invasive testing.
Instead, imagine a future where we can harvest cells from a cancer patient, for instance, and create hundreds of miniature models of their disease in a matter of minutes.
These personalised disease models could then be grown and treated with a range of potential therapies to identify the most effective option – all before the patient ever receives a single injection.
This vision is driving the next phase of our research, where we’ll be collaborating with Peter MacCallum Cancer Centre to bring this concept closer to reality.
Our work not only aims to advance our understanding of how these miniature models can predict treatment outcomes but to also help pave the way for more effective, patient-specific therapies in the fight against cancer and other diseases.