Breaking the skin barrier
These chemically engineered polymer-tantalum devices may be the key to tricking skin to grow on implants, opening the way for implantable robotics
Two years of snowboarding, professional kitesurfing and hanging out on the beach isn’t most people’s idea of how medical research breakthroughs are made.
But for Dr Gil Stynes, taking time out from surgical training may have revolutionised the potential for body implants and opened the way for wires, robotics, and even decorations, to be permanently implanted through our skin.
Watching crustaceans at the beach got Dr Stynes thinking about the possibilities for mimicking an exoskeleton in humans by creating medical devices that could attach through the skin without the risk of infection.
Intravenous lines, catheters, and prosthetic rods are all implanted through the skin but are at constant risk of infection because our skin refuses to adhere to them, leaving a gap open to bacteria.
It is a fundamental barrier to the development of permanently implanted robotics to replace lost limbs and for reconstructing body parts with biomaterials such as metals and plastics.
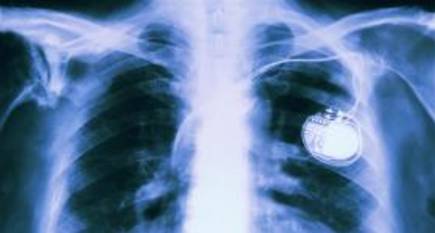
Part human, part robot: The future of medical implantables
But nine years on from when he first started thinking about creating human exoskeletons, Dr Stynes has taken an exciting step towards cracking the problem.
A small pilot study on four pigs suggests skin can be made to grow onto an ingenious polymer and tantalum structure embedded into the skin without causing infection.
It means that the scaffold-based structure can potentially seal off the body from infection while leaving a channel for a device to be inserted such as a catheter, a wire, or a prosthetic rod drilled into bone.
“One of the holy grails of biomaterials research has been working out a way to get skin to grow onto and attach to metals and plastics without the risk of infection.
It looks like this design and technique may have solved the problem,” says Dr Stynes, who is researching his PhD at the University of Melbourne. “It could pave the way for fully implantable robotics, prosthetics, catheters, intravenous lines, and the reconstruction of surgical defects with artificial materials.”
Professor Richard Page, Director of Orthopaedics and the Centre of Orthopaedic Research and Education at Barwon Health and Deakin University, said the ability of the scaffold to make the skin think it was growing on other skin is potentially a major finding.
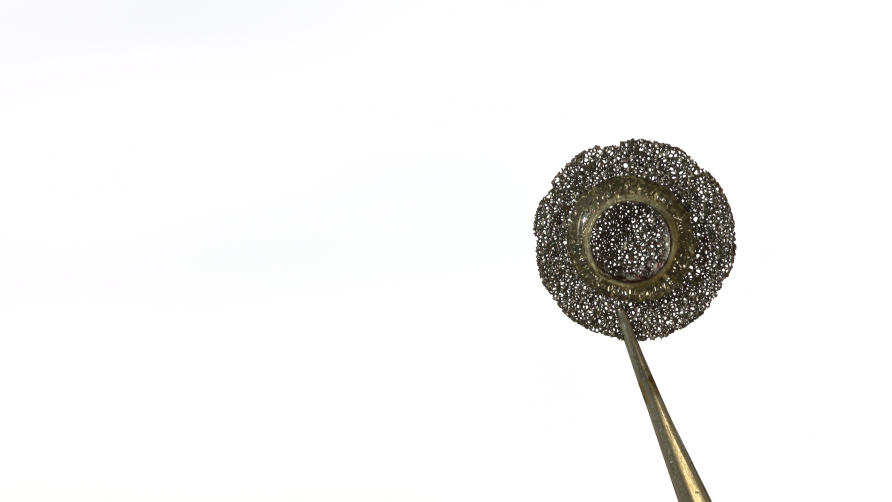
“If the pilot study results can be validated more broadly, and eventually on human subjects, then the potential flow on benefits are huge, because the skin/implant interface is an ongoing problem,” Professor Page says.
Dr Stynes’ research has combined medical science with advanced materials science, physics and chemistry to create a patented design that he hopes could ultimately be manufactured locally.
Based at the University of Melbourne’s Department of Surgery at St Vincent’s Hospital in Melbourne, where he is undertaking his PhD, Dr Stynes has done much of the research work at University Hospital Geelong in partnership with Deakin University and the Commonwealth Scientific and Industrial Research Organisation.
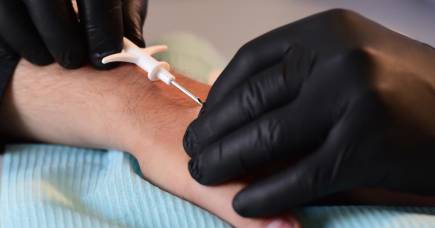
Four myths about insertable tech and why they're wrong
Skin implants and infection
The results of the pilot study have been published in the US-based Journal of Biomedical Materials Research, and while too small to be statistically significant, the qualitative results are encouraging and have opened the way for a larger trial.
The study found that after a month skin appeared to attach to the structure with no signs of inflammation or infection.
The issue with skin implants has always been that, rather than attaching to the biomaterial, the skin’s outer layer, called the epidermis, grows down around it in a process called marsupialisation that leads to the risk of infection.
“Infections in skin-penetrating medical devices are seen every day in our hospitals,” says Dr Stynes.
Lines inserted into veins are at high risk of becoming infected after just a few days. For already weakened patients, such as those with cancer or someone in intensive care, an infection in the blood stream can be disastrous.
Aside from the human toll caused by infection, according to a US study, one infected intravenous line in the neck or groin can cost the community US$25,000 in treatment and extended care.
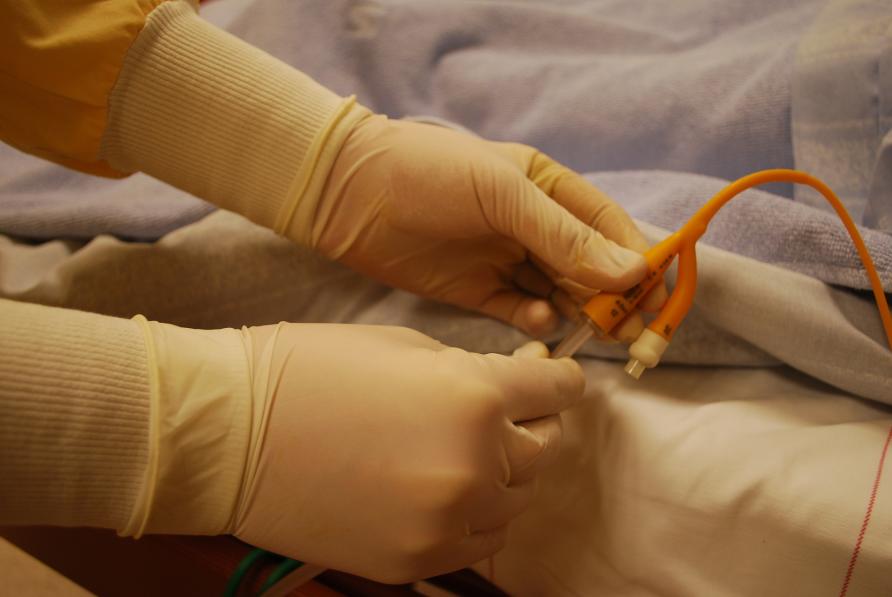
The problem of infection at the interface between skin and biomaterials is why reconstructive surgery remains reliant on tissue brought from other areas of the body.
“These operations can be very traumatic for the patient,” says Dr Stynes.
“Today we routinely put metals and plastics into the body and they work well, as long as they are covered by tissue. For example, many people have had bones repaired with titanium plates and screws, hip replacements, artificial heart valves, or hernias repaired with plastic mesh.
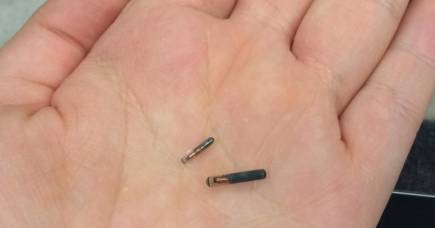
Superhuman abilities could lurk under your skin
“But there is no reason why we couldn’t reconstruct surgical defects completely with biomaterials if the skin-biomaterial interface is robust and infection-free.”
Implanted titanium rods are increasingly being used to attach prosthetics in a technique called osseointegration, but marsupialisation persists and infection rates have been reported as high as 18 per cent.
“If we could implant an osseointegrated rod without the risk of infection, we could run wires and other conduits through the rod and attach robotic devices, knowing the patient will be fine,” he says.
Tricking our skin
The structure designed by Dr Stynes is made from a material that looks very similar to interconnected honeycomb, and was developed by Melbourne-based biotech Cytomatrix Pty Ltd, which contributed seed funding to the research.
Dr Stynes attached a doughnut-shaped cap to the scaffold and implanted the whole structure into skin, aided by a surgical technique called negative pressure wound therapy.
This involves using a vacuum pump to stretch tissue, draw out fluid from the wound, and increase blood flow to the area, all of which promotes healing. The vacuum helps the tissue to integrate throughout the scaffold.
Finally, Dr Stynes worked with CSIRO to chemically bind collagen, a skin protein, to the scaffold in a bid to “trick” the skin into treating the device as if it were other skin. While further testing is required to confirm the findings, the pilot study suggests the skin appeared to be fooled.
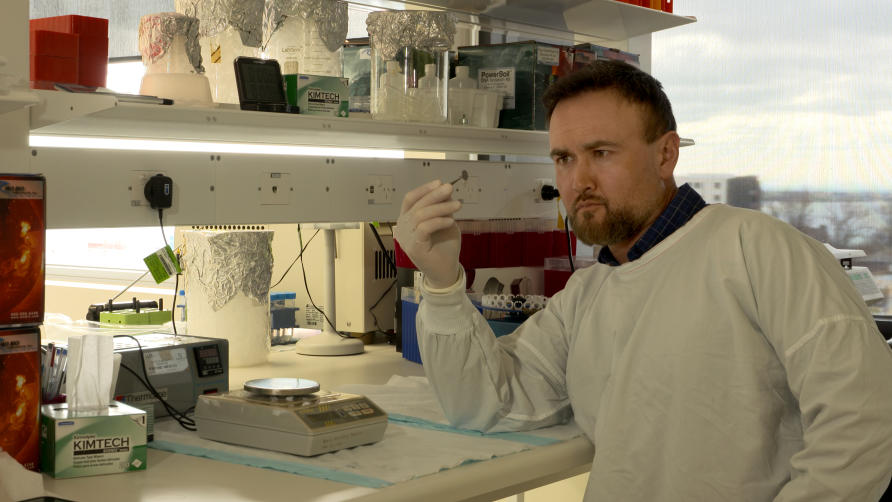
“The key to this technique is having all the elements working in concert – the negative pressure wound healing, the design of the cap-scaffold, and a reactive surface with chemically attached collagen,” says Dr Stynes.
One of Dr Stynes’ supervisors, Professor Wayne Morrison, a plastic surgeon and former head of the Department of Surgery at St Vincent’s Hospital, University of Melbourne, says the results are encouraging and well worth pursuing.
“Getting skin to bond to foreign material has been extremely difficult and this three-pronged approach is novel and very interesting,” Professor Morrison says.
Dr Stynes’ research has been support by grants from both the Commonwealth’s Advanced Manufacturing Cooperative Research Centre and Victoria State’s Victorian Centre for Advanced Materials Manufacturing.
“This project has only been possible by bringing together private industry, universities, federal and state governments, hospitals, and CSIRO to create a device that could be really beneficial to patients worldwide and that could be a boost for the Australian biotech industry.”
Banner: Paul Burston, University of Melbourne