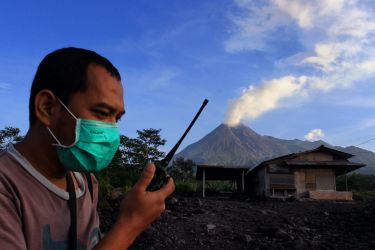
Sciences & Technology
The dual risks of natural disasters and COVID-19
The 2016 magnitude 6.0 earthquake in the Petermann Ranges gives researchers key insights into how some faults may break in Australia to produce larger earthquakes
Published 4 June 2020
On average, Australia experiences a magnitude six or larger earthquake every 8.5 years.
Australia’s vastness and highly concentrated population centres reduce our exposure to most of these earthquakes, like the July 2019 magnitude (Mw) 6.6 earthquake that occurred far beneath the Indian Ocean about 300 kilometres west of Broome in Western Australia.
However, our seismic risk is not insignificant, and learning about the conditions that control the location and severity of our earthquakes is a critical part of evaluating our exposure to this hazard.
The magnitude 5.6 Newcastle earthquake in 1989 that claimed 13 lives and caused an economic loss of $A4 billion exemplifies the potential risk Australian cities are exposed to even if a moderate earthquake occurs near them.
Sciences & Technology
The dual risks of natural disasters and COVID-19
The largest on-shore Australian earthquake in the last 20 years, a magnitude of 6.0, occurred on 21 May 2016 around 3:45 am (local time) in a remote desert region of Central Australia near the Petermann Ranges.
But what caused Central Australia’s old, strong, and cold cratonic crust to break and produce this significant earthquake?
Our research provides new insights into how weak rock layers embedded in the strong Australian crust may have played a role in setting off this rare earthquake.
Agencies like Geoscience Australia and the United States Geological Survey estimated the magnitude and approximate location of the 2016 Petermann earthquake within hours.
The shallow hypocentre (that sits within the uppermost five kilometres of the crust) suggested a surface rupture was probable and led us to rapidly deploy our earthquake response team to carry out an earthquake ‘near-field investigation’.
Together with Geoscience Australia, we also rapidly installed 10 seismometers in the epicentral region to record aftershocks. These locations could be used to determine the subsurface geometry of the fault plane.
Despite a major desert storm severely hampering field work, our geologists scoured the land for evidence of a surface rupture, both on foot and using a drone. They eventually located evidence of the rupture two weeks into their field work.
As a result, we were able to map the deformation associated with a 21 kilometre long trace of a surface rupture in detail – where the ground had up lifted with a maximum vertical displacement of one metre.
Data collected from this earthquake near-field investigation allowed us to determine the physics of the fault rupture associated with the Petermann earthquake, helping us to understand Australia’s seismic hazard.
Following a major earthquake, smaller aftershocks occur on ‘sticky’ patches in and around the fault plane that didn’t fail completely in the mainshock. Locating these aftershocks allows seismologists to map out the geometry of the fault plane that slipped during the mainshock.
Analysis of aftershocks indicated the Petermann earthquake was caused by rupture of a triangular fault plane dipping at about 30˚ to the northeast.
Based on seismic data analysis and in situ observations, it was evident that the fault slipped with a thrust sense of motion. We also estimated the fault area to be between 124 kilometres² and 158 kilometres².
This contradicted an initial hypothesis that the Petermann earthquake may have activated the Woodroffe Thrust – a major geologic weak zone dipping to the southwest – located about 10 kilometres to the northwest of the estimated epicentre.
Interestingly, some aftershocks were aligned with the Woodroffe Thrust, suggesting that it may have been seismically activated following the mainshock, possibly through a mechanism known as Coulomb stress transfer.
This is a geologic mechanism that changes stress conditions in the surroundings of an earthquake, either bringing nearby faults to or away from failure.
Stress drop, which is an average measure of the amount of stress relieved during fault rupture can be estimated using seismological methods.
Australian earthquakes typically have high stress drop estimates, resulting from the brittle breaking of strong rocks that can store large amounts of elastic strain energy; this is the energy that’s stored in rocks as they compress, stretch or shear due to tectonic forces.
The Petermann earthquake was different. It yielded a stress drop less than 10 per cent of the Australian average.
This, together with a smooth fault plane dipping at a 30˚ angle, as indicated by the alignment of aftershocks, suggested the Petermann earthquake was sourced from the rupture of a weaker fault.
Revisiting our geologic field maps of the earthquake near-field, we found mylonitic layers rich in mechanically weak micaceous minerals oriented subparallel to the estimated fault plane.
This provides evidence for lower rock friction across the fault plane that controlled the seismologic characteristics of the Petermann earthquake.
Although rocks in the Australian cratonic crust are typically characterised as strong, our results show that rock strength varies from one place to another, resulting from millions to billions of years of accumulated tectonic deformation.
Sciences & Technology
Earthquakes that talk to each other
In regions where weaker rocks are present, earthquakes may rupture faults under low friction.
The vast majority of global earthquakes occur at depths inaccessible to human observation. So, establishing a link between geologic structure at fault-scale and earthquakes is difficult.
However, the Petermann earthquake is a rare example where we’ve been able to link earthquake formation with pre-existing geologic structure by combining seismological modelling and geological field mapping.
With this insight, geologic maps, including those of rocks beneath our urban centres, have an added benefit of enabling us to forecast the likely ruptures of future earthquakes and better understand the degree of Australia’s seismic hazard.
Banner: A false-colour image with the West MacDonell Ranges in the upper section of the image and part of the Petermann Ranges in the lower section/European Space Agency