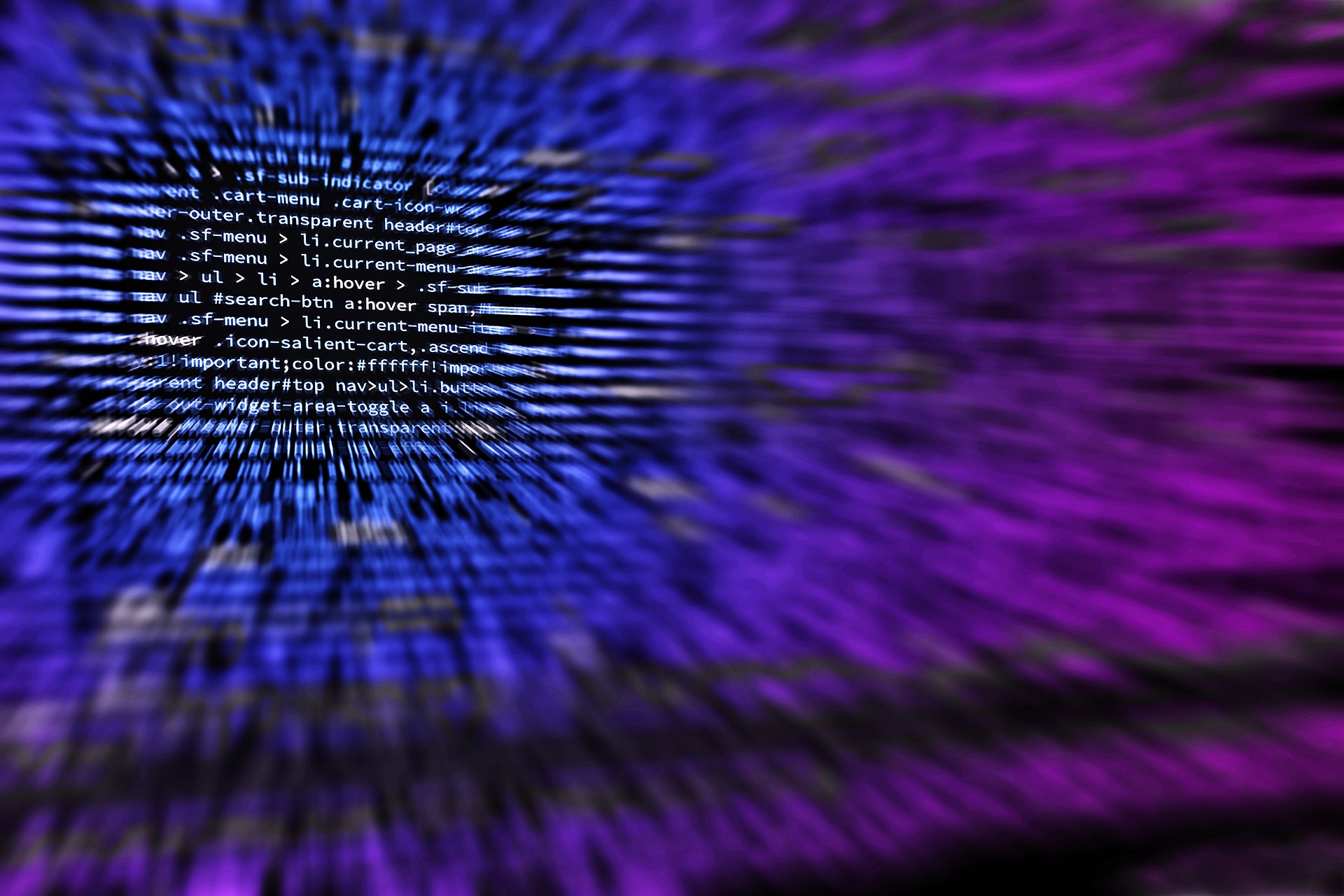
Sciences & Technology
Q&A: How algorithms are fighting epilepsy
Miniature immature organs in dishes, known as organoids, may hold the key to major breakthroughs in treatments for epilepsy and autism, as well as a range of other diseases
Published 6 November 2015
Epilepsy researcher Professor Steve Petrou and developmental neuroscientist Associate Professor Mirella Dottori discuss the potential of organoids, an exciting new avenue of medical research.
These lentil-sized balls of cells can only live for a few months, but they are allowing researchers to study biology in action outside a patient’s body. And they could change how we treat some very serious conditions.
“Many epilepsy drugs are targeted at the symptoms and not the cause. We’re going after the cause with our organoid research,” says Professor Petrou.
Sciences & Technology
Q&A: How algorithms are fighting epilepsy
Banner image: Neurons on a scaffold, taken using a Helium Microscope. Image: Dr Babek Nasr, University of Melbourne Centre for Neural Engineering
Subscribe to Up Close through iTunes.