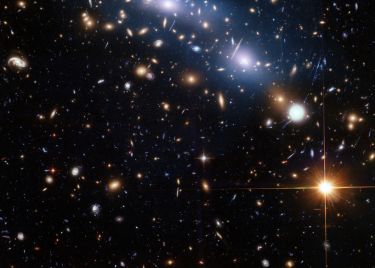
Sciences & Technology
Finding dark matter in the dark
From watching the heavens to discovering waves of light, relativity and entropy, understanding the nature of time has been a major human endeavour
Published 2 January 2020
In the beginning there was Day, that fundamental unit most simply determined by the rising and setting of our sun.
Humanity went on to track the seasons, the cyclical passage of which came to be recognised as the Year, and we noted the 28-day cycle of the phases of the moon, marking the beginnings of what we now know as Months.
Soon, the more sophisticated of the ancients observed that a year could be marked by the rising of certain stars and constellations that returned to the same position about every 365 days.
The passage of time – at least, how humankind has defined, tracked and measured it – has become ever more complicated, says Professor David Jamieson at the School of Physics at the University of Melbourne.
Sciences & Technology
Finding dark matter in the dark
“Eventually, astute observers noticed there were even longer cycles, namely the procession of the equinoxes (when twice a year the visible sun lies directly over the equator).
“The earth’s spin doesn’t always point in the same direction, it wobbles a little, which means the seasons gradually slip through the year.”
For example in 13,000 years, Australia’s winter will have flipped to occur in January and its summer in July.
“The first calendars tried to make order out of all these random numbers and the Romans eventually made the year 365 days, which was actually a little too short,” says Professor Jamieson. “It’s actually 365 and a quarter in round figure terms – so every four years we have to stick in an extra day and have a leap year.”
In fact, the year is closer to 365.24 days – or 365.242196, according to the US National Institute of Standards and Technology – which means we need to miss out a leap day every 100 years.
Similarly, the way in which we have carved up the months doesn’t bear any close resemblance to the phases of the moon. Instead we decided it is more convenient to have 12 months made up of mostly 30 or 31 days each, with only one month of 28 days – or 29 in leap years.
Sciences & Technology
The frontiers of physics: From planets to photons
As life got busier, more precise time-keeping was developed. The day was divided into hours, minutes and seconds. And time zones were introduced, originally to make railway timetables work.
Nowadays, notes Professor Jamieson, the best atomic clocks would only lose a few hundred seconds over the 14 billion year age of the universe!
But while we have long tried to carve up time, it is a concept that even history’s greatest geniuses have struggled with.
Galileo, observing the swinging of an incense burner in church, discovered that the time it took to complete one oscillation, or period, was independent of how high it swung. It is why pendulums became the basis for accurate clocks.
It led to Galileo’s First Relativity Principle, determining that the laws of physics didn’t depend on absolute motion.
As Professor Jamieson points out, the Earth orbits the Sun with a speed of over 100,000 km/h, but we are entirely oblivious to this speed because everything moves together.
“Galileo’s relativity principle tells us we can’t detect this speed by doing physics experiments sealed up in a lab on Earth. Instead, we have to look outside towards the Sun to start to detect it,” says Professor Jamieson.
Sciences & Technology
Four important things that this picture tells us
“In the same way you can safely pour a drink whether on a speeding aircraft or when the aircraft is parked at the gate.”
Isaac Newton extended Galileo’s principle, postulating that the universe is governed by a majestic clockwork (as Jacob Bronowski has described Newton’s thought) where all clocks everywhere at all times tick in perfect synchronisation.
But, as Professor Jamieson points out, “Newton didn’t know anything about electro-magnetism.”
James Clerk Maxwell, in the 19th century, and Albert Einstein, in the 20th, certainly did. Maxwell demonstrated that light is a wave with electrical and magnetic components and travels at one billion km/h. Einstein further deduced from Maxwell’s equations that the speed of light was the same regardless of your state of motion and, as a result, formulated his theory of relativity.
In essence according to Einstein’s theory, there is no majestic synchronicity, instead everything is moving relative to everything else.
Time therefore doesn’t pass at the same rate for everyone. If you observed a fast-moving clock, you will measure its time passing more slowly than your own time. Essentially, because of this “time dilation”, the watch of the fast-moving observer ticks slower than the watch of the stationary observer. And vice versa.
Health & Medicine
The meaning in our stars
Einstein also showed that gravity affects time – the stronger the gravity, the slower the clock. Clocks deep in the gravitational field of a star or planet will be observed from outer space to tick slowly.
The most practical demonstration of this can be seen in today’s global positioning system (GPS) satellites. Because they are moving so fast above the earth, time dilation means their atomic clocks are slowed by 6 millionths of a second per day compared to identical clocks on the surface. But simultaneously the less gravity speeds up the orbiting clocks by 45 millionths of a second per day when measured from the surface of the Earth.
The net effect then is that the clock on the satellites are 39 microseconds a day faster, which would translate into a positioning error of about 12 kilometres. Scientists correct this by factoring into their algorithms this General Relativistic Effect – or, as Professor Jamieson puts it, “flicking the switch to Einstein-On”.
One other vital truth about time is that it only goes forward – that is, from the past to the future. What gives it this property is known as the Arrow of Time. To scientists it is more prosaically called the Second Law of Thermodynamics, which says energy will always dissipate into disorder – entropy.
In lectures, Professor Jamieson illustrates this with a time-lapse, infra-red video of a cup of coffee cooling, dissipating its energy into his office.
“We went from a low entropy scenario, where all the energy was concentrated in the cup… to a high entropy state, where its energy gets shared around the atoms all over the room.”
Sciences & Technology
A brief history of Hawking’s scientific legacy
Relativity and entropy are what’s driving the universe, says Professor Jamieson.
“Until the Big Bang, there was no time, only space. There was no ticking, no passage of here and now into the future, until something happened,” he says.
From an almost infinitely low entropy state, in a tiny fraction of a second what became the universe suddenly expanded and continues to do so. It’s massive but finite energy is transforming into an ever-more disorganised, high entropy form.
“Time isn’t an innocent bystander against which you conduct the affairs of your life or even the laws of physics,” says Professor Jamieson.
“Time is intimately tied up with the structure of space and the way things work. It’s not a blank canvas upon which you impose your existence, it’s part of your machinery and existence – the passage of time.”
Banner: Getty Images