Chasing the secrets of the universe
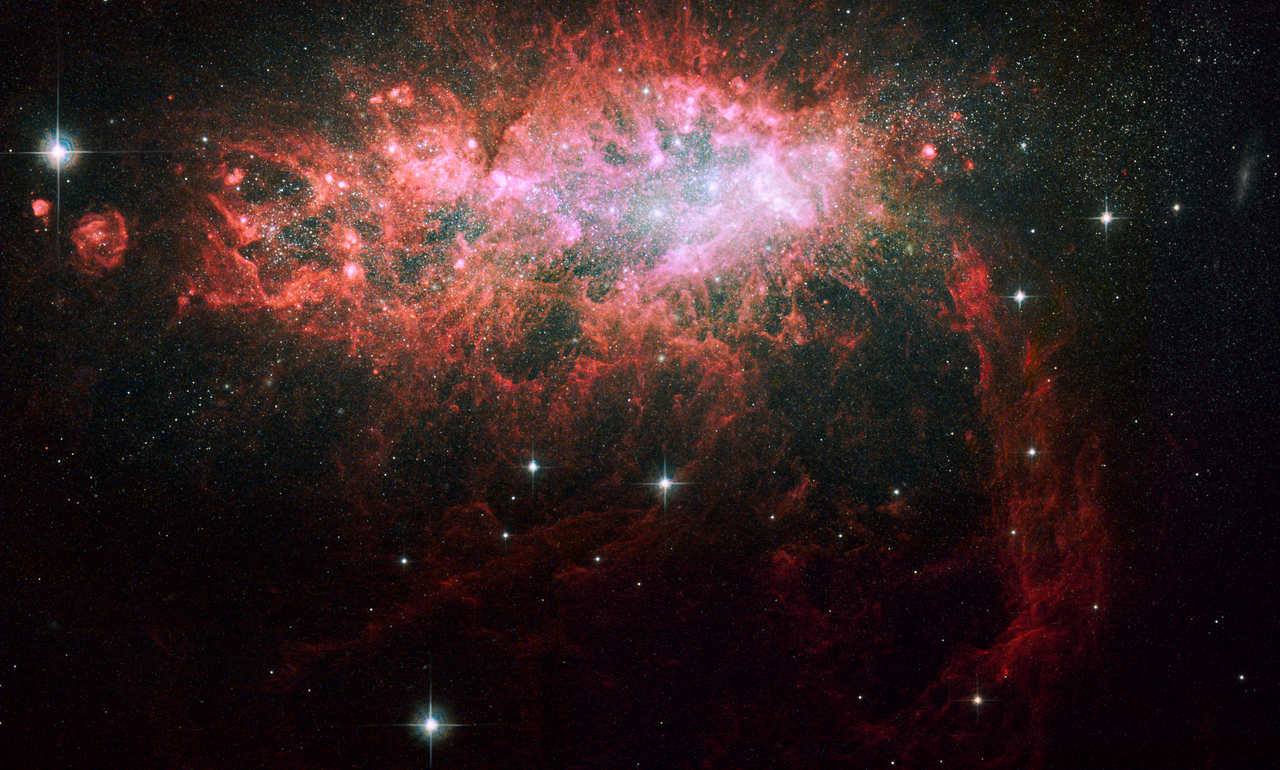
Rising star and physicist Phillip Urquijo, winner of the Eureka Prize Emerging Leader in Science award 2015, is working on some of the remaining puzzles in fundamental physics
Published 24 September 2015
When I was young I was always obsessed with maths and physics, and science magazines, New Scientist, Scientific American. I would read New Scientist every week. I just liked facts – scientifically proven evidence of how something works. In primary school, I became obsessed with space and I did a science project on how the solar system worked. From that point I was hooked. I thought I wanted to be an astrophysicist – later it was nuclear and, finally, particle physics.
At uni I read the Nobel Prize-winning Leon M Lederman’s The God Particle. A disagreeable title to most physicists, but it was about the history of discovery of new particles and the search for the Higgs boson. It described the life of an experimental particle physicist, working on accelerators and detectors. I was completely captured, did my PhD on the Belle experiment in Japan, then went on to work in Europe and Japan on other large-scale particle physics experiments.

It’s the big questions in physics that have always interested me. The project I’m working on now, Belle II, is seeking answers to some of the remaining puzzles in fundamental physics. It’s about, in part, understanding why the universe as we see it pretty much has no antimatter left in it.
The Standard Model of physics tells us that at the beginning of the universe, when things went from a state of pure energy to the existence of matter, that antimatter and matter would have been produced 50/50, they would have existed in equal abundance.
By antimatter I mean anti-atoms: atoms that are made up in the same way as regular atoms but the electric charge is reversed. When matter meets antimatter, it annihilates, it forms pure energy. So we may not exist if antimatter and matter were still around in the same quantities.
To use an analogy, say you were to meet an anti-person, and you shook their hand, you would both annihilate – you wouldn’t want to do this!
The fact that we don’t see any antimatter left, and we have no mechanism to understand why, is a pretty fundamental question of physics. It goes to the heart of why we exist fundamentally. There must have been a difference in behaviour between anti-matter and matter, and that difference could be really, really small but it’s enough to have wiped out the antimatter in the universe at this point.
There are 650 people working on the experiment. The accelerator, the SuperKEKB, which is 3km round, is located in the countryside about 70 kms out of Tokyo. Electrons and positrons will be sent round long tubes via magnets and accelerator cavities that bring them close to speed of light. They will travel round the circuit, positive in one direction, negative in the other, and collide in the detector, Belle II.
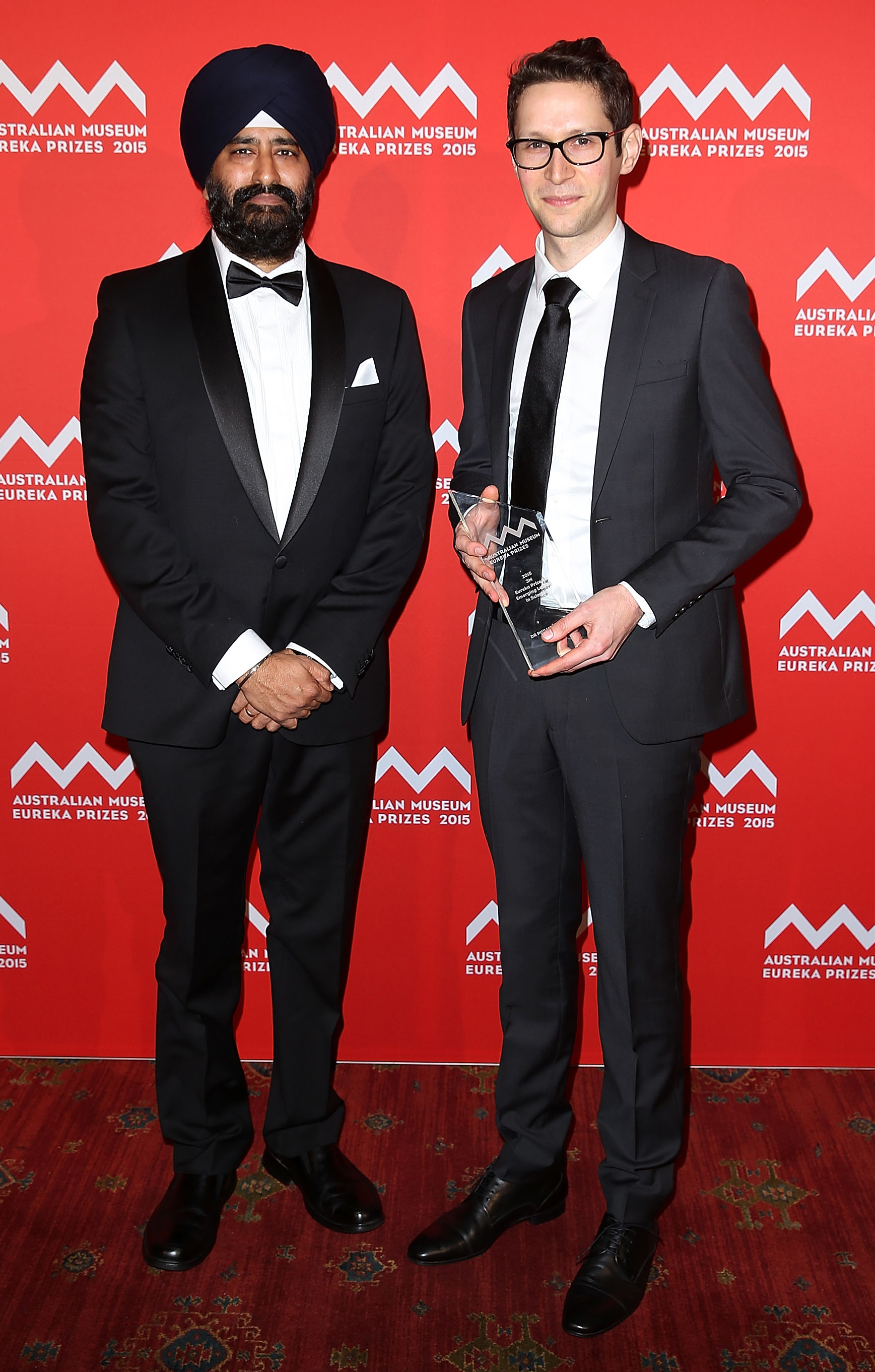
Our Japanese partners are responsible for the particle accelerator, and the University of Melbourne is partially responsible for the detector, Belle II, and a key component is being constructed right here, downstairs in David Caro building. From January 2016, we will endeavour to make the highest number of collisions of particles by any machine ever built in an accelerator. After a period of rigorous testing, from 2017, the detector, Belle II will be used, and will run for the next eight years.
Every two nanoseconds bunches of electrons and positrons will collide in Belle II. We’re hoping to see something here that explains the disappearance of antimatter, as well as a range of other questions. It could be a new particle; it could have been something that existed in a tiny, tiny fraction after the big bang.
My job is to prepare the experiment for all the different kinds of data analyses for the next ten years: to assess what observables and what measurements are required, build focused international teams, define what we’re looking for, and prepare our software, our algorithms, for finding rare processes within our huge data set. We will produce 100 billion so-called “beauty”-quarks that we’ll study, but also hundreds of billions of other things at the same time. So we may be looking for only a handful of what’s produced. It’s a needle in haystack problem!
Exploring the fundamental questions in physics is essentially about the pure pursuit of knowledge, the search for something that’s unknown, but of course there will be applications for the technology.
This is going to produce one of the biggest data sets ever from a scientific experiment, so we have to use a global scientific computing grid, and people working on this are developing technologies for global computing for multiple applications – it’s setting the model for big data. Another application relates to real time image processing. Because the data-taking is so high rate, with collisions every two nanoseconds – essentially like taking a digital photo every two nanoseconds and interpreting it very quickly – this technology could be used for the development of bionic eyes.
If we did find a new particle, or something that would lead to solving one of these puzzles it would no doubt be ‘big’. The biggest recent discovery was the Higgs boson. But in a way if we saw something, it would be bigger, because the Higgs boson had been predicted. If we find something, it won’t have been predicted, so it will be a huge leap.
– As told to Michelle Moo
Banner image: In this gorgeous Hubble Space Telescope image, two massive star clusters – youthful counterparts to globular star clusters in our own spiral Milky Way galaxy – are seen left of centre. NASA/public domain.