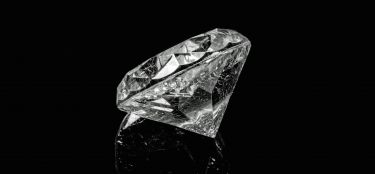
Sciences & Technology
Spinning diamonds for quantum precision
New research shows that when it comes to measuring the force between two atoms - it may actually be hiding from you
Published 12 December 2018
In 1687, Sir Isaac Newton published his comprehensive theory of gravity – the force that is essential for keeping us from floating off into space – and since then, our understanding of forces has focused right down to nanoscale and atomic forces.
So you can get a sense of scale, atoms are around a tenth of a nanometre in size, or a million times smaller than the width of a human hair.
Three hundred years after Newton’s theory, in 1986, scientists started to measure atomic forces using the aptly-named Atomic Force Microscope (AFM), pioneered by Nobel Prize Winner Professor Gerd Binnig along with Professor Calvin Quate and Professor Christoph Gerber.
“The AFM is widely used in nanoscience and nanotechnology because of the range of applications that it can benefit. All materials are composed of atoms and so, by understanding the forces related to them, we can learn more about their structure and properties,” says Professor John Sader from the University of Melbourne’s School of Mathematics and Statistics and the ARC Centre of Excellence in Exciton Science.
Sciences & Technology
Spinning diamonds for quantum precision
The AFM uses a small cantilever beam (its length is the same as the width of a human hair) to feel the shape of a surface and sense the forces it encounters — in much the same way the stylist of a record player operates. A sharp tip at the cantilever’s end interacts with the surface.
In its widely used ‘dynamic mode’, the AFM operates just like a woodpecker: its small cantilever beam has a sharp tip attached to it, and when the cantilever oscillates up and down, its sharp tip feels the surface. This then changes the oscillation of the cantilever.
Once this change in oscillation is measured, the force exerted between the atoms is extracted using a mathematical algorithm developed in 2003 by Professor Sader and Professor Suzanne Jarvis from Trinity College Dublin — called the Sader-Jarvis method - which is now widely used in the AFM.
“But then, out of the blue, my colleague Professor Franz Giessibl from the Institute of Experimental and Applied Physics at the University of Regensburg in Germany emailed me some new experimental data which showed weird behaviour that no one had ever seen before,” says Professor Sader.
These measurements were performed by Ferdinand Huber, who is Professor Giessibl’s PhD student.
When measuring the force between two atoms, Mr Huber and Professor Giessibl observed that different mathematical algorithms to extract the force gave different results. They were surprised because many researchers had checked this over the past two decades and had seen no anomaly.
So, what was going on?
“I set about testing the various algorithms and exploring options with my colleague, mathematician Professor Barry Hughes — what we found totally surprised me.
Sciences & Technology
A big discovery in a tiny package
“It turned out that some force laws occurring in nature give good results while others give garbage – for example, instead of getting a smooth and continuous force (the correct result), you can actually get an (incorrect) messy discontinuous and jumpy force. But then the question was ‘why’?”
This problem had effectively been hiding in plain sight from the entire scientific community.
“After studying it for a while, the issue turned out to be mathematically subtle. Force laws that belong to something called Laplace space – which everyone has tested – are fine. It’s the ones that aren’t part of this space that cause the problem – and there are many of these in nature.”
By looking at the details of this subtlety, Professor Sader was able to identify the cause of the issue and formulate a new mathematical theory and method that identifies when it arises in a real measurement, allowing an AFM practitioner to avoid it.
“I like to think of our discovery as giving practitioners the ability to see a ‘pot hole’ in the road ahead, and so avoid it with no damage. Previously, this pot hole had gone unnoticed and drivers were sometimes steering straight into it,” Professor Sader says.
The next step is to try and understand how to remove this ‘blind spot’ or ‘pot hole’ entirely.
“Our work also highlights the importance of mathematicians and experimentalists working together to solve an important technological problem. Without both of these skill sets, this problem would not have been identified and solved. It had gone unnoticed for more than 25 years.”
Professor Sader says this new understanding may provide insight into the operation of other dynamic AFM force measurements by identifying a previously unexplored feature.
Banner: Getty Images