Trapping malaria in a gene net
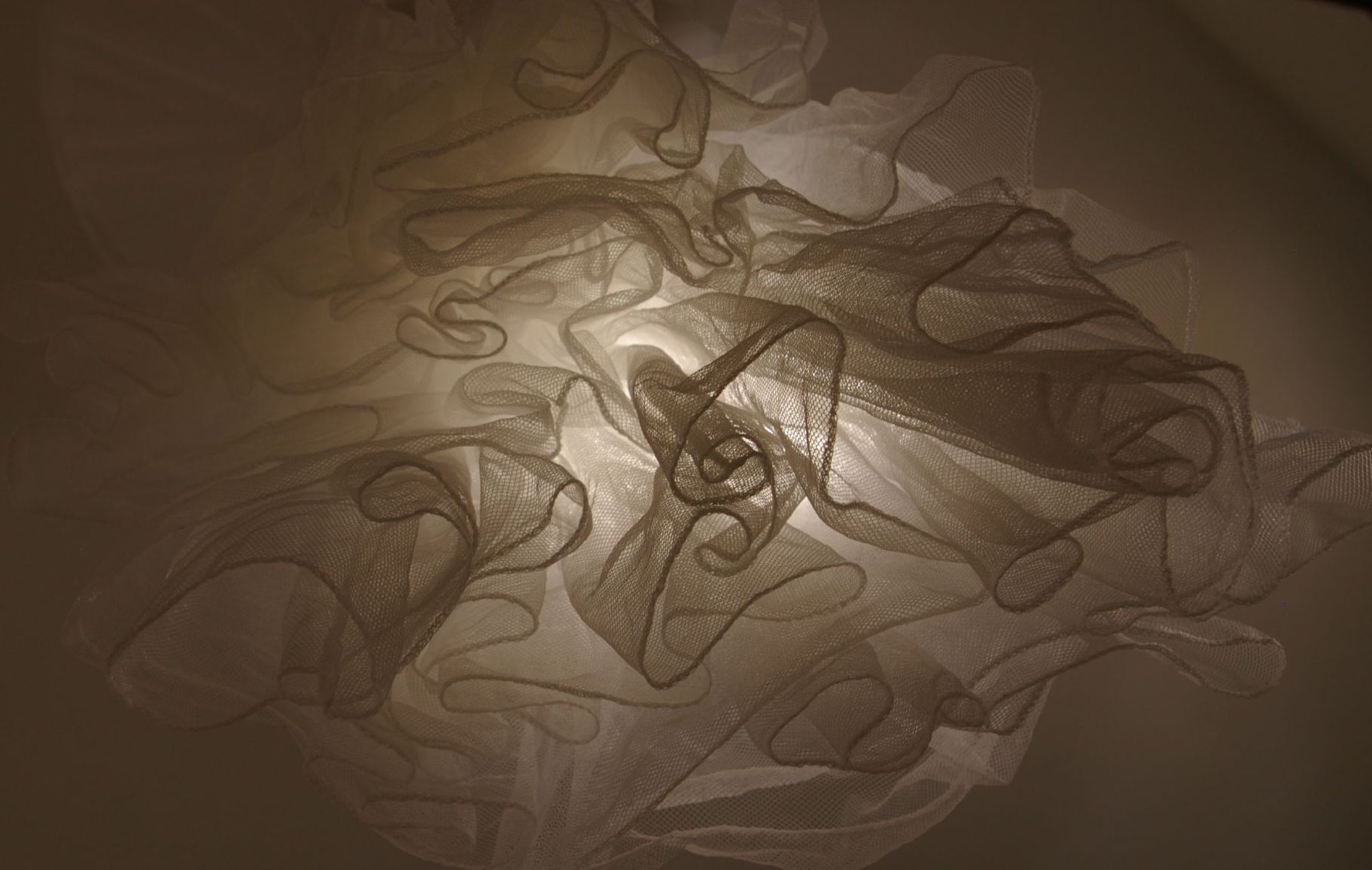
The ability of this disease to mutate to nullify drugs is actually its downfall, delivering a new weapon for stopping the spread of resistance
Published 15 April 2016
Malaria parasites are too clever for their own good – fatally.
In what may be a game-changer in the battle against malaria’s ability to dodge new drugs by building resistance, scientists have discovered how to turn the parasite’s own mutations against it, making it impossible for the mutated parasite to survive in mosquitoes.
It is a giant step towards controlling malaria because the parasites, clever as they are, won’t be able to spread their adapted resistance from person to person.
The discovery, published in US journal Science, also means an existing anti-malaria drug, atovaquone, previously thought too prone to resistance is in fact safe to be used on a mass scale.
Malaria remains one of the world’s biggest health challenges killing up to 635,000 people every year, most of them young children in Africa.
An international team led by scientists from the University of Melbourne has used basic biology and genetic analysis to discover that when the malaria parasite mutates to resist atovaquone, the mutation makes it impossible for the parasite to subsequently survive when it gets inside a mosquito.
As a result the parasite dies before it can reach the mosquito’s salivary glands and be passed back into another human where it would spread its drug resistance.
“Genetically it is a very neat little trap,” says Professor Geoff McFadden, at the University of Melbourne’s School of Biosciences, who jointly led the research with colleagues Dr Dean Goodman and Dr Josephine Siregar, from Indonesia’s Eijkman Institute.
“The parasite’s resistance strategy is also its Achilles’ heel. The mutation is a quick and easy fix for the parasites to get around the drug, but they are digging a hole they can’t get out of.”
Anti-malarial drug developers should now be able to incorporate the same genetic “trap” in new drugs to prevent resistance spreading. Atovaquone came off patent in 2013.
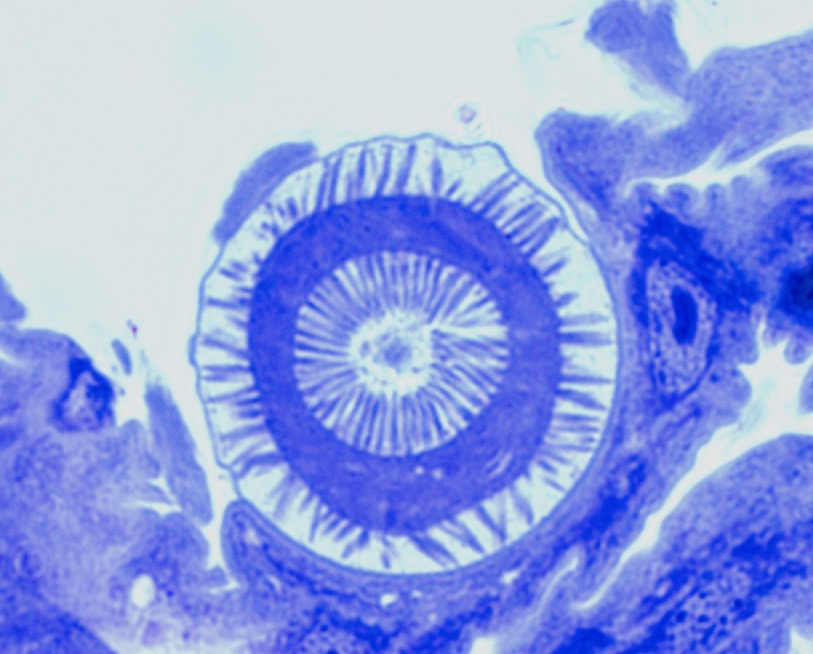
“This will address the drug resistance problem because it gives us a new strategy to manage it,’’ Professor McFadden says.
The use of atovaquone as an anti-malarial drug has always been handicapped by the ability of malaria parasites to develop resistance. In 2000, pharmaceutical company Glaxo Smith Kline brought out a patented version of the drug that was safe for key risk groups such as young children and pregnant women and marketed it as Malarone.
Genetic mutation
Professor McFadden says that because of the high risk of resistance spreading, Malarone was specifically targeted at the travellers’ market rather than for mass administration in areas of Africa and Asia where malaria is endemic. It was consequently priced at about 10 times the cost of other anti-malarial drugs. But until now no one had thought to examine whether the atovaquone-resistant parasites could even survive in the mosquitoes that spread malaria. It turned out they couldn’t.
“It is bizarre that no one had looked before now, but it was simply assumed that the resistance could be spread just like other drug resistance,” Professor McFadden says.
The origin of the discovery was a conference in Jakarta in 2013 when scientists, including Professor McFadden, and colleagues from Australia, Indonesia, Japan and the US compared notes on their work on malarial resistance. They realised the genetic mutation in response to atovaquone should make it difficult for malaria to survive in mosquitoes. Tests by Dr Goodman, Dr Siregar and Vanessa Mollard, using a rodent strain of the malaria parasite in Professor McFadden’s lab, confirmed it couldn’t survive once inside the insect.
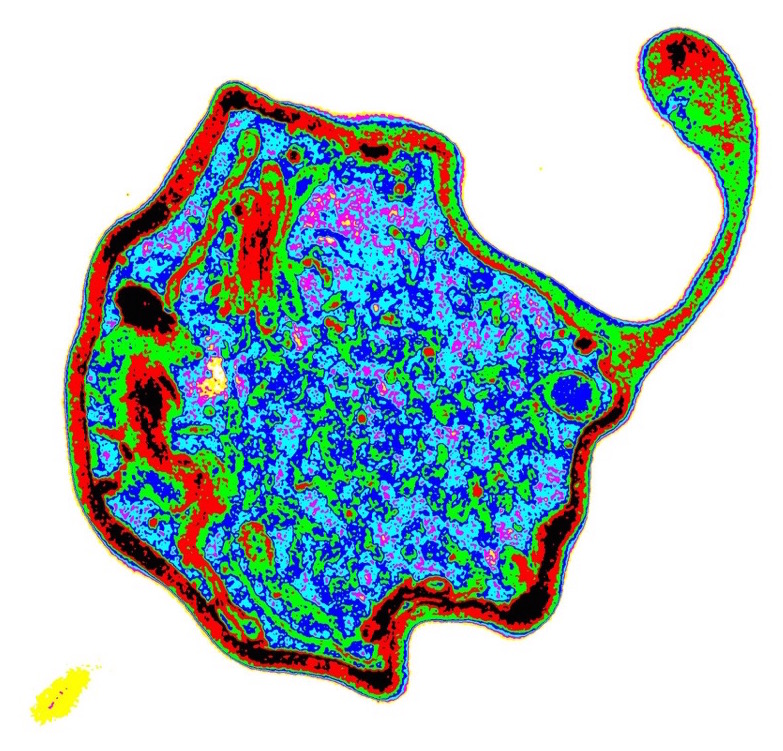
The results were then replicated when a human strain of the drug resistant parasite was fed to mosquitoes in the US lab. “It was the Eureka moment,” Professor McFadden says.
The human body is a very accommodating environment for parasites, but the mosquito is a much harsher one. The problem for the drug-resistant malaria parasite is that in the human body the parasite can readily cope with the biological cost of living with atovaquone – its respiration becomes less efficient – but it becomes a fatal defect inside the mosquito.
“In humans the parasite is living in an incredibly rich environment with plentiful amounts of glucose and protein and a regulated environment with a steady temperature. It is like a land of milk and honey. But when it moves into the insect where temperatures drop and resources are scarce, it has to work a lot harder,” Professor McFadden says.
The task now is to conduct field trials in Africa where blood from people suffering from the drug-resistant malaria parasite will be tested in mosquitoes to confirm that the resistance can’t be passed on. If that proves successful, then new generic versions of atovaquone could be used on a mass scale to effectively treat and prevent malaria.
Artemisinin is currently the best available anti-malarial drug compound for mass administration, but resistance has developed in Cambodia and Thailand and is spreading. Professor McFadden said atovaquone drugs could be used to manage outbreaks of resistance to artemisinin.
“This isn’t going to eradicate malaria, but it will help to control it,” he says. “To get rid of malaria we need not only good drugs but also a vaccine, which so far is proving difficult to develop, and mosquito controls. There isn’t just one solution.”
The discovery included work by researchers from Indonesia’s Eijkman Institute and Hasanuddin University, Japan’s Jichi University, Nagasaki University and Tokyo University, and in John Hopkins University in the US.
Multi-media content: Paul Burston
Banner Image: A mosquito net. Picture: Andrea Kirkby/Flickr